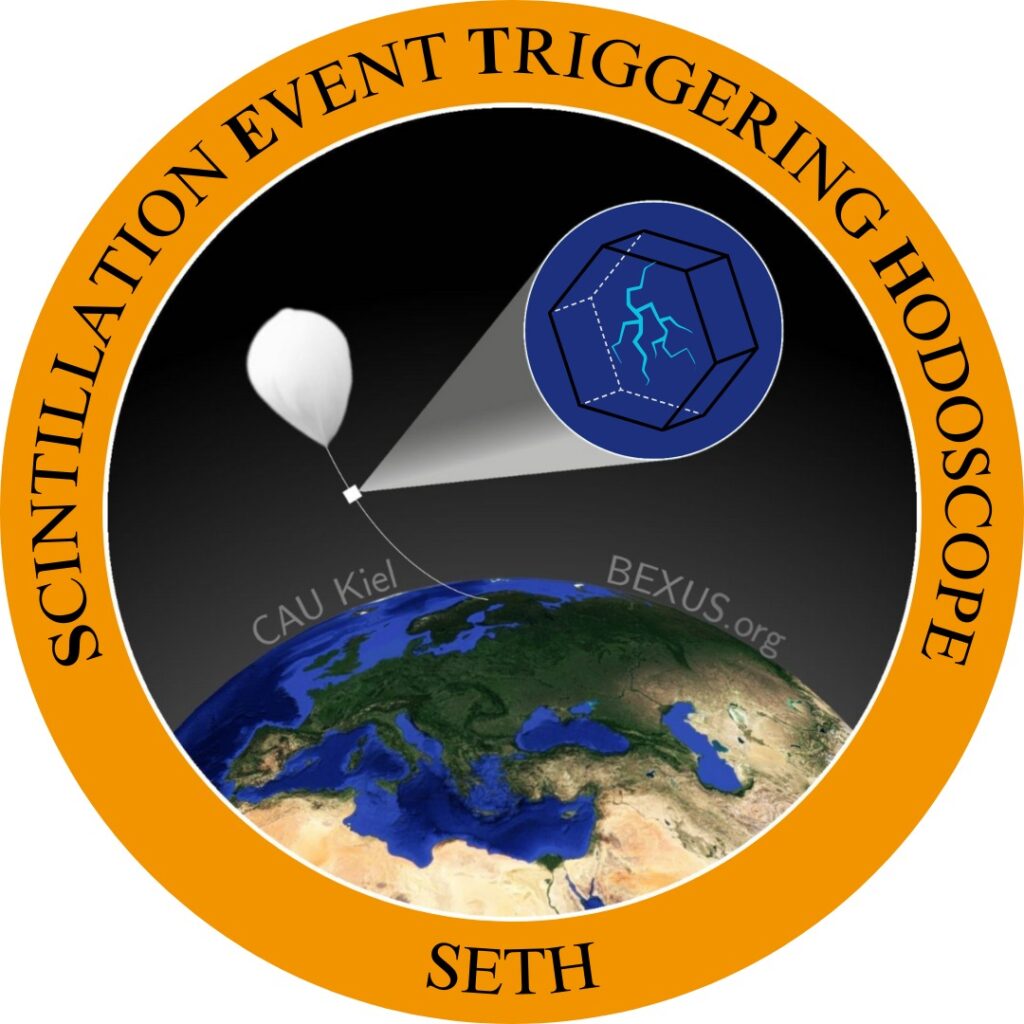
SETH or the Scintillation Event Triggering Hodoscope is a detector bulit by a student group from Kiel University. We plan to take part in the BEXUS (Balloon EXperiments for University Students) cycle 16 in autumn of 2025. For this, we have submitted an application and will know by the end of the year whether we are chosen or not.
The acronym already says quite well how the device works. We will be using two sets of photodiodes and two large BGO scintillation crystals to measure the angle of incidence of galactic cosmic rays hitting our atmosphere at a height of more than 20 km. To do this, we detect the energy loss of a single particle in all five detector stages to determine what particle species it is. By reading out which detectors saw the particle we can calculate its angle.
Triggering in this case means that only the particles are recorded that lose energy in the BGO crystal.